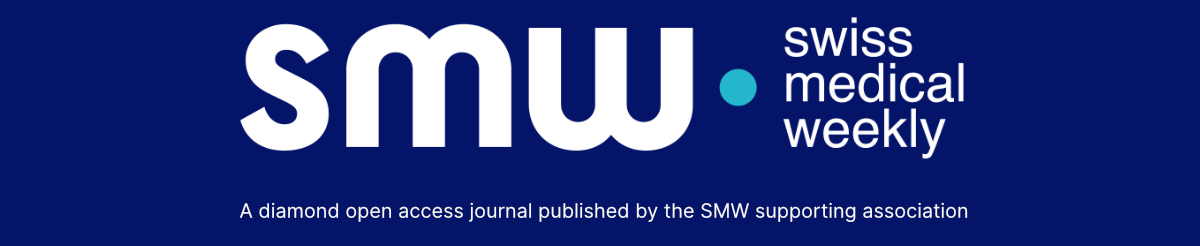
Tumour-infiltrating myeloid-derived suppressor cells (MDSCs) are a heterogeneous population of myeloid cells. The main feature of MDSCs is their ability to suppress T-cell activation and function, which leads to immunosuppressive activity in the tumour microenvironment. Higher numbers of circulating and tumour-infiltrating MDSCs have been observed in a large number of patients with various types of tumour, and are linked to poor prognosis, especially in hormone-driven tumours. Recently, it has been demonstrated that the recruitment of MDSCs in prostate cancer confers resistance to canonical endocrine therapies, opening a new approach to the treatment of hormone-driven cancer patients.