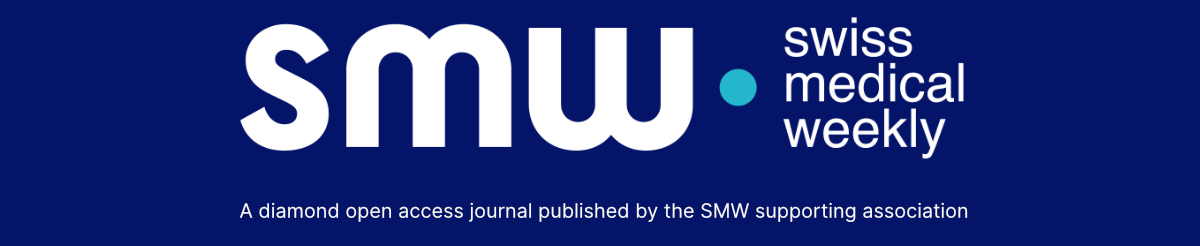
In chronic cardiomyopathy, mechanical circulatory support plays an increasingly important role for children as the shortage of suitable donor hearts increases times on the transplant waiting list. Ventricular assist devices (VADs) for adults have evolved dramatically over the last decade, both as a bridge to transplantation and for permanent support. In contrast, VADs designed for children, especially for all age groups, are still in their infancy. The Medos HIA and the Berlin Heart Excor are specially designed for children with a body surface area <1.2 m2. Increased experience with existing paediatric VADs and the introduction of third-generation VADs for the paediatric age group offer new possibilities for children suffering from end-stage heart failure. We review the literature on this topic, summarise the indications and contraindications for long-term support VADs and describe the decision-making algorithm used at our institution for use of long-term VADs in children.